Protein-DNA Interactions in the Epigenetic Machinery
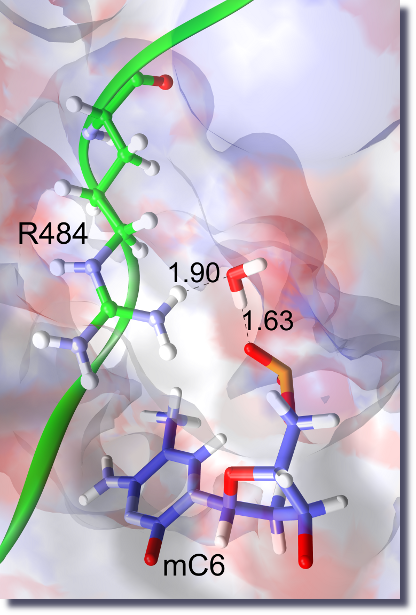
Every cell in an organism (like the human body) contains the same genetic information in its DNA sequence.
Nevertheless, cells from different tissues look different and perform different functions (thus, have different phenotypes).
How does a particular cell know then which genes, out of the entire genetic code, to express?
In addition, this information has to pass from parent cell to daughter cells.
From what is known up to now, chemical modification (methylation) of certain cytosine bases and post-translational modifications of histone proteins form the code which regulates the expression of genes (known as the 'epigenetic code').
How this is done at the molecular level, especially the recognition between the modified groups and the DNA or proteins involved, is the subject of several of our projects.
Caterina Bianchi and Ronen Zangi, Base-Flipping Propensities of Unmethylated, Hemimethylated, and Fully Methylated CpG Sites, J. Phys. Chem. B, 117, 2348-2358 (2013)
Caterina Bianchi and Ronen Zangi, UHRF1 Discriminates Against Binding to Fully-methylated CpG-Sites by Steric Repulsion, Biophys. Chem., 171, 38-45 (2013)
Caterina Bianchi and Ronen Zangi, How to Distinguish methyl-Cytosine from Cytosine with High Fidelity, J. Mol. Bio., 424, 215-224 (2012)
Ronen Zangi, Ana Arrieta and Fernando P. Cossío, Mechanism of DNA Methylation: The Double Role of DNA as a Substrate and as a Cofactor, J. Mol. Biol., 400, 632-644 (2010)
Computer-Aided Drug Design
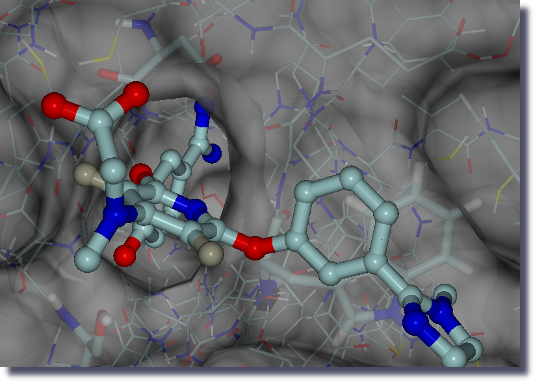
In recent decades, the performance of many tasks has been optimized with the help of computer modeling.
Today, this is only partially the case for designing drugs.
The objective in this case is to fine-tune the interactions between a target receptor (protein) and a ligand (drug) through the evaluation of the corresponding binding affinity.
In practice, differences in binding affinities (between the ligand and other related ligands) are calculated by constructing thermodynamic cycles based on rigorous statistical mechanics formalism.
The advantage of using computers for this type of design is the ability to employ unphysical pathways to describe the change from one ligand to the other (computational alchemistry).
Nevertheless, the results are physically meaningful.
In this project, we apply state-of-the-art techniques for interesting systems.
Furthermore, we also aim to improve these currently employed approaches via enhancing the sampling of configuration space.
Salts and Cosolute Effects
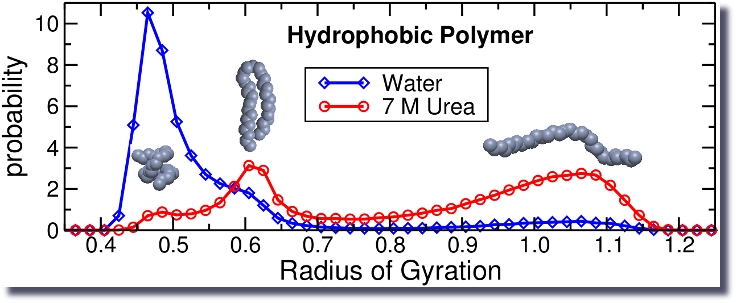
If you have a molecule solvated in solution, you can change its properties by adding certain cosolutes.
One example where this effect is well known is related to proteins.
You can greatly increase, or decrease, the solubility of proteins by adding specific type of salts.
The ranking of the ions to induce this effect is known as the Hofmeister Series or as the salting-in/salting-out ability of the ions in solutions.
Another example of a cosolute effect is the ability of urea (or other denaturants) to destroy the native structure of proteins.
It is trivial to seek the understanding of the effect these cosolute induce in terms of the cosolute or the cosolute-water system.
However, we found that this is not possible and the changes observed are functions of the ternary solute-cosolute-water system.
In fact, the best descriptor that quantify the magnitude of the induced changes is given by the preferential binding parameter.
Ronen Zangi, Can Salting-In/Salting-Out Ions be Classified as Chaotropes/ Kosmotropes?, J. Phys. Chem. B, 114, 643-650 (2010)
Ronen Zangi, Ruhong Zhou and B. J. Berne, Urea's Action on Hydrophobic Interactions, J. Am. Chem. Soc., 131, 1535-1541 (2009)
Ronen Zangi, Morton Hagen and Bruce J. Berne, Effect of Ions on the Hydrophobic Interaction between Two Plates, J. Am. Chem. Soc., 129, 4678-4686 (2007)
Hydrophobes and Solvent-Induced Interactions
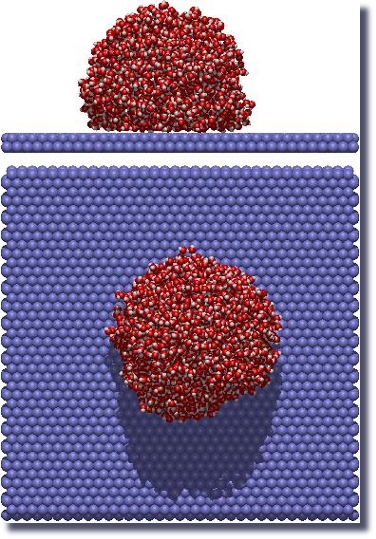
Oil and water do not mix and, therefore, when you put a drop of water on an oily (hydrophobic) surface, the drop will maintain its shape and will not spread on the surface.
This phenomenon originates not because the molecules forming the oil phase (hydrophobic particles) repel the water molecules.
On the contrary, they attract them!
What is then the origin of the hydrophobic effect? It all comes down to the strong propensity of the water molecules to associate with each other.
However, this is not so simple as evident by the fact that the mechanism responsible for the attraction between two hydrophobic particles depends on the size of these hydrophobes.
For small hydrophobes, the driving force is purely entropic, whereas for large hydrophobes it is both entropic and enthalpic with a ratio determined by the character of the hydrophobe.
Recently, we studied the origin of these driving forces and characterized them for graphene sheets.
In addition, the behavior of these solvent-induced interactions and the associated drying transition have been investigated as a function of temperature.
Ronen Zangi, Driving Force for Hydrophobic Interaction at Different Length Scales, J. Phys. Chem. B, 115, 2303-2311 (2011)
Ronen Zangi and Bruce J. Berne, Temperature Dependence of Dimerization and Dewetting of Large Scale Hydrophobes: A Molecular Dynamcs Study, J. Phys. Chem. B, 112, 8634-8644 (2008)